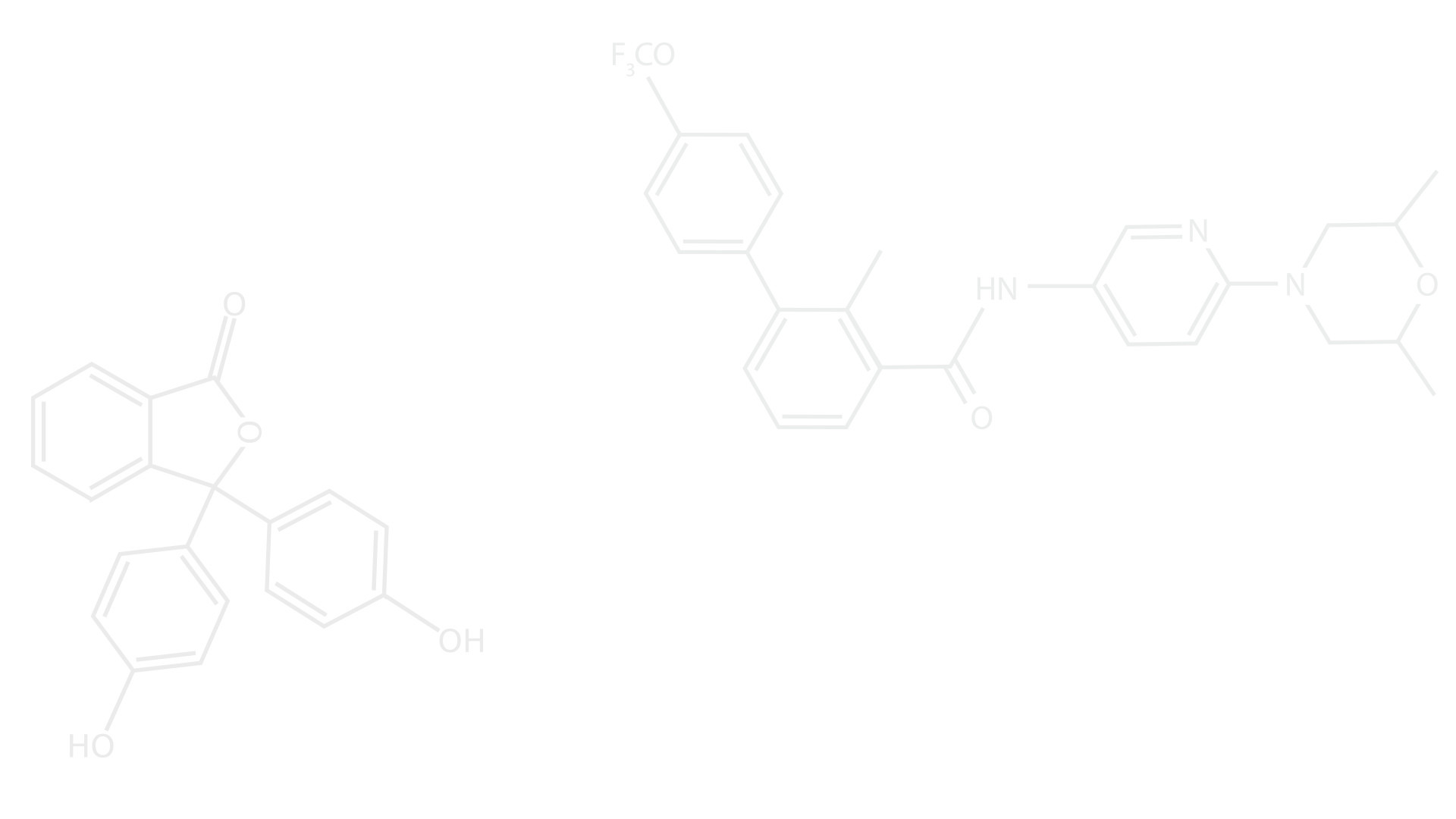
RESEARCH
Post Doctoral Experience
My research has focused on developing multiphoton technologies to study the structure and function of biological systems spanning from cerebral organoids, to small animals with ultrashort pulses.
In the first project, I developed a custom-made three-photon microscope and optimized the laser and microscope parameters so that we can perform fluorescent and label-free three-photon microscopy in awake mice. Below, three photon dual color Ca+2 (green) and blood vessel (magenta) imaging is shown below to monitor the activity of V1 neuron populations across the all cortical layers in vivo with a single cell resolution while a sinusoidal grating is presented to the animal.
Our measurements of physiological responses and tissue-damage thresholds define pulse parameters and safety limits for damage-free three-photon imaging. We image functional visual responses of neurons expressing GCaMP6s across all layers of the primary visual cortex (V1) and in the subplate. These recordings reveal diverse visual selectivity in deep layers: layer 5 neurons are more broadly tuned to visual stimuli, whereas mean orientation selectivity of layer 6 neurons is slightly sharper, compared to neurons in other layers. Subplate neurons, located in the white matter below cortical layer 6 and characterized here for the first time, show low visual responsivity and broad orientation selectivity.
Our measurements of physiological responses and tissue-damage thresholds define pulse parameters and safety limits for damage-free three-photon imaging. We image functional visual responses of neurons expressing GCaMP6s across all layers of the primary visual cortex (V1) and in the subplate. These recordings reveal diverse visual selectivity in deep layers: layer 5 neurons are more broadly tuned to visual stimuli, whereas mean orientation selectivity of layer 6 neurons is slightly sharper, compared to neurons in other layers. Subplate neurons, located in the white matter below cortical layer 6 and characterized here for the first time, show low visual responsivity and broad orientation selectivity.
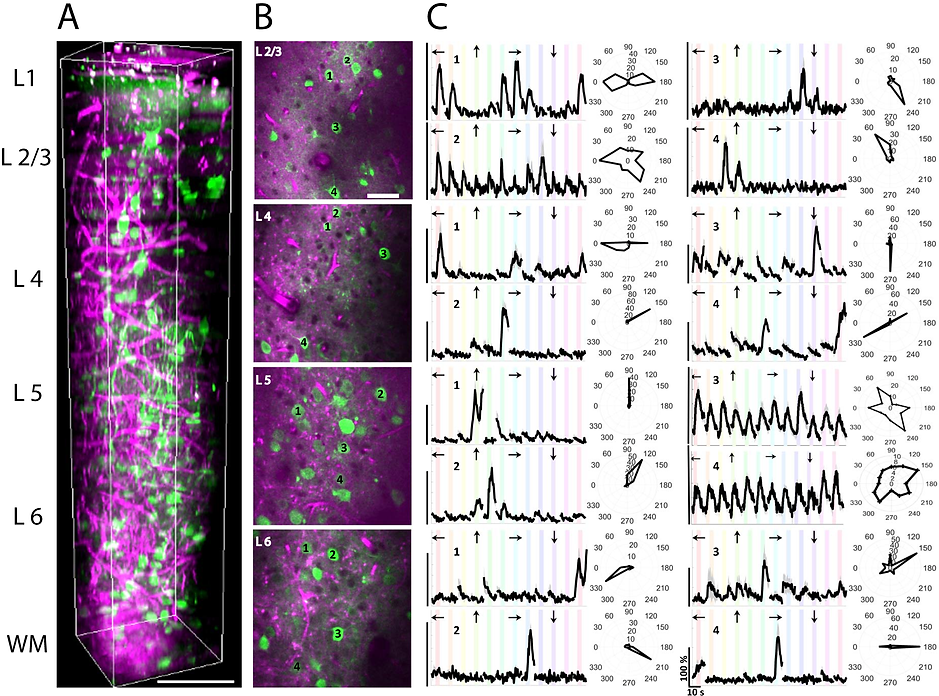
Characterization of visual responses of different layers in V1 of awake mice. (a) Three-dimensional rendering of a sequence of 450 lateral three-photon images acquired with 2-μm increment. Green color represents GCaMP6s signal, and magenta color represents label-free THG signal generated in the blood vessels in the visual cortex and myelin fibers in the white matter. Scale bar, 100 μm. (b) Selection of lateral images from layer 2/3, 4, 5, and 6. Scale bar, 50 μm. Field of view in all lateral images is 250 μm. (c) Average calcium responses (ΔF/F) for representative cells in each layer over 10 trials in response to oriented gratings moving in specific directions (arrows above each trace) and their orientation tuning curves in polar plots. Discontinuities in (ΔF/F) traces are due to the randomization of each stimulus direction in each trial. (ΔF/F) scale bars correspond to 100% and time scale bar corresponds to 10 s as shown in the bottom right panel.
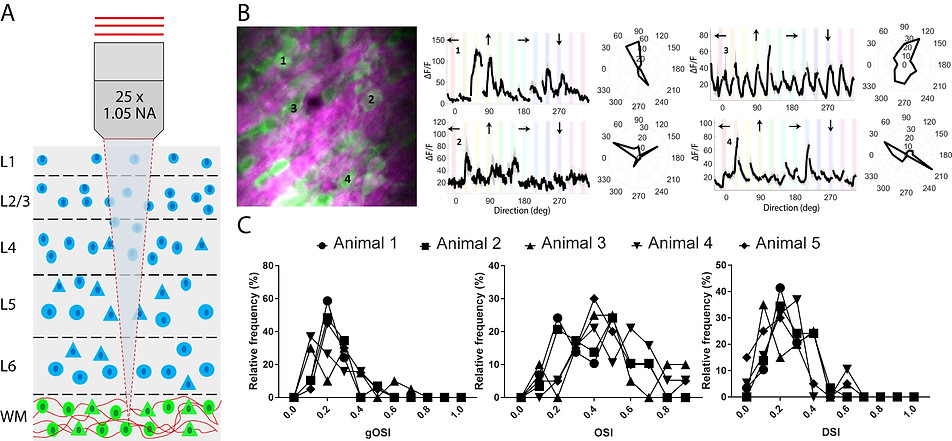
Characterization of visual responses of subplate neurons in the white matter below V1 layer 6. (A) Schematic coronal section of V1 showing locations of cortical (blue) and subplate (green) neurons surrounded by bundles of myelinated axons (red). (B) Left: Three-photon image of subplate neurons (green) and myelinated axons (magenta) in the white matter. Scale bar, 50 μm. Right: Average calcium responses (ΔF/F) for representative cells in response to oriented gratings drifting in specific directions (arrows above each trace) and their orientation tuning curves in polar plots. Discontinuities in (ΔF/F) traces are due to the randomization of each stimulus direction in each trial. (ΔF/F) scale bars correspond to 100% and time scale bar corresponds to 10 s as shown in the bottom right panel. (C) Population responses of subplate neurons showing global OSI, local OSI, and DSI.
In the second project, I combined three laser wavelengths in order to perform two-color two-photon microscopy and one-photon optogenetics. Cortical neurons often respond to identical sensory stimuli with large variability. However, under certain conditions, the same neurons can also respond highly reliably. The circuit mechanisms that contribute to this modulation, and their influence on behavior remains unknown. Here we used novel double transgenic mice, dual-wavelength calcium imaging and temporally selective optical perturbation to identify an inhibitory neural circuit in visual cortex that can modulate the reliability of pyramidal neurons to naturalistic visual stimuli. Our results, supported by computational models, suggest that somatostatin interneurons (SST-INs) increase pyramidal neuron reliability by suppressing parvalbumin interneurons (PV-INs) via the inhibitory SSTPV circuit. Using a novel movie classification task, we further show that, by reducing variability, activating SST-INs can improve the ability of mice to discriminate between ambiguous stimuli. Together, these findings reveal a novel role of the SST-PV circuit in modulating the fidelity of neural coding critical for visual perception.
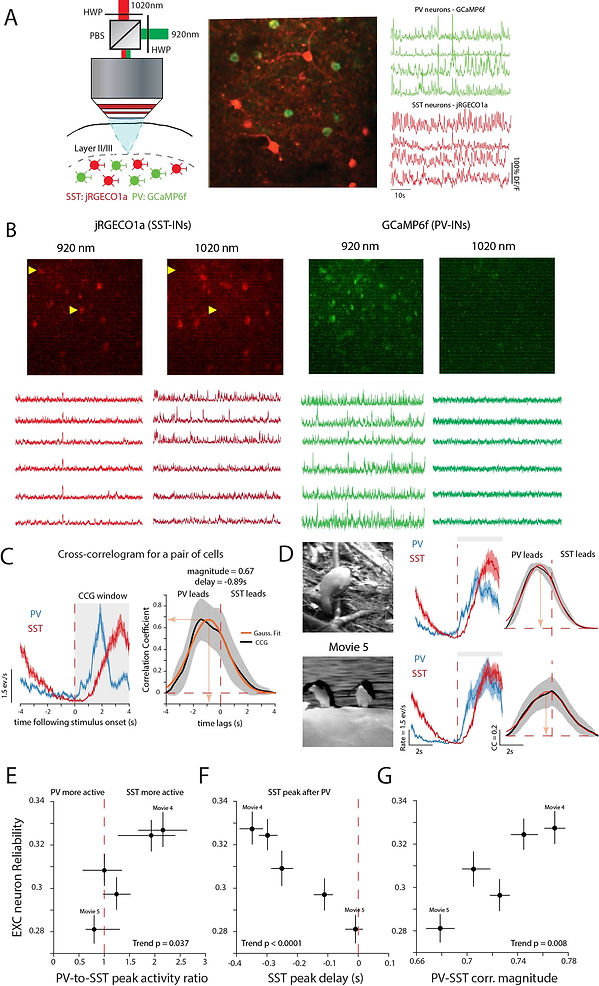
SST-INs are temporally delayed relative to PV-INs in reliably processed movies (a) Left: Experimental setup. Briefly, a 1020nm laser and a 920nm laser were combined using a half wave plate (HWP) and a polarizing beam splitter (PBS) to optimally activate jRGECO1a and GCaMP6f in SST and PV-INs respectively (see Methods). Middle: Example field of view showing co-labeled PV and SST-INs. Image covers a cortical area of 150µm x150µm. Right: Example calcium transients from simultaneously recorded interneurons. (b) Left: Trial-averaged responses from a pair of simultaneously recorded PV and SST-INs. Right: Cross-correlogram (CCG) of this pair. Orange line shows Gaussian fit to trial averaged CCG. Shaded areas, SEM over trials. (c) Difference in correlation for two different movies for the same PV and SST-IN pair. (d-f) More reliable movies have a stronger SST peak activity compared to PV (d), longer delays between SST and PV peak activity (e) and stronger PV-SST correlation at peak delay (f). Data are from 2292 pairs, 5 mice. Data points denote median ± 95% CI for each movie. Pvalues computed using F-test to measure significance of the trend relative to a constant model.
In the third project, I extended the first project to measure effective attenuation lengths (EALs) in primary and higher visual areas in awake mouse brain. The structure of brain regions is assumed to correlate with their function, but there are very few instances in which the relationship has been demonstrated in the live brain. This is due to the difficulty of simultaneously measuring functional and structural properties of brain areas, particularly at cellular resolution. Here, we performed label-free, third-harmonic generation (THG) microscopy to obtain a key structural signature of cortical areas, their effective attenuation lengths (EAL), in the vertical columns of functionally defined primary visual cortex and five adjacent visual areas in awake mice. EALs measured by THG microscopy in the cortex and white matter showed remarkable correspondence with the functional retinotopic sign map of each area. Structural features such as cytoarchitecture, myeloarchitecture and blood vessel architecture were correlated with areal EAL values, suggesting that EAL is a function of these structural features as an optical property of these areas. These results demonstrate for the first time a strong relationship between structural substrates of visual cortical areas and their functional representation maps in vivo. This study may also help in understanding the coupling between structure and function in other animal models as well as in humans.
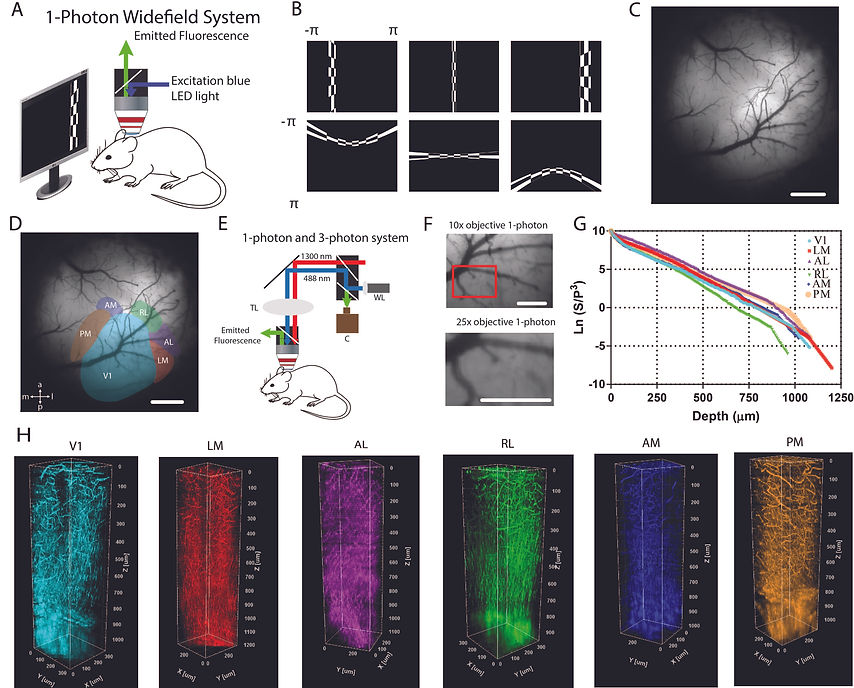
(A) One-photon imaging system to obtain retinotopic maps of visual areas. Blue light was used to excite GCaMP6s in the brain of awake head-fixed mice. Emitted light (green) was collected by the camera. (B) Vertical and horizontal bars (containing flickering black and white checkerboards, and corrected for screen distance and curvature) were drifted across the screen and used to map visual areas of the cortex. (C) Blood vessel architecture of an example mouse with cranial window in the right hemisphere. (D) Representation of six cortical visual areas overlaid on the blood vessel architecture. (E) Combined oneand three-photon system to perform depth-resolved THG imaging. (F) Low magnification (10x) and high magnification (25x) images of V1 obtained with one-photon imaging system coupled with three-photon imaging system. (G) Semi-logarithmic plot for ratio of PMT signal (S) and cube of laser power (P) with respect to imaging depth for third harmonic generation (THG) imaging. Slope of this curve provides EAL values for the cortex and for the white matter. As the slope increases (decreases), EAL value decreases (increases). (H) Three-dimensional rendering of a sequence of >200 lateral THG images acquired with 5-µm increment. THG signal is generated in the blood vessels in the cortex and is generated in myelin fibers in the white matter. Each color represents a different visual area. Scale bars present 1 mm in (C-D), and 0.5 mm in (F).
PhD Experience
My PhD research was based on understanding the interaction between ultrashort pulses and the tissue in terms of nonlinear imaging and ablation to resolve a clinical problem, vocal fold scarring. Vocal fold scarring is one of the predominant causes of voice disorders, affecting an estimated 2-6 million people in the US alone. Unfortunately, current methods for treating vocal fold scarring are inconsistent and frequently ineffective, and there is currently no accepted treatment for restoring phonation to scarred vocal folds. In order todevelop a reliable technique/solution to vocal fold scarring , I focused my attention to develop table-top and endoscopic systems and optimize laser parameters accordingly.
First, we demonstrated creation of sub-epithelial voids with nonlinear imaging guidance using a compact, turn key fiber laser. The ablation parameters, including pulse duration, pulse energy, spot size, and scanning speed are comparable to the specifications of our recently developed miniaturized femtosecond laser surgery probes, illustrating the feasibility of developing an ultrafast laser surgical instrument.
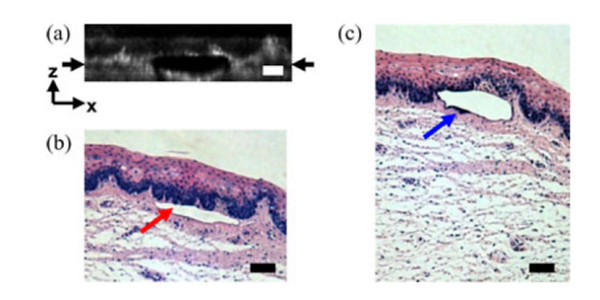
Histological follow-up of voids created in porcine vocal fold with 500 nJ pulses. (a) Cross-sectional second harmonic generation image of a void ablated approximately 100 µm beneath the tissue surface. Black arrows denote the plane targeted for ablation. (b) Follow-up histology of the void shown in (a). Red arrow indicates sub-epithelial void created by ultrafast laser ablation. (c) Histology section of a different void in the series. Blue arrow indicates small section of epithelial nuclei that were separated out of the epithelium during ultrafast laser ablation. Note the thicker void and the thinner epithelium in comparison with (b). Histology images are stained by H&E. All scale bars are 50 µm
Second, we performed a comprehensive study of quantification of laser parameters including laser ablation threshold, bubble life time for ablating scarred tissue samples. In addition, we presented a new method for extracting tissue-specific ablation threshold and scattering lengths of different tissue layers. Overall, this study shed light on the required laser parameters for successful implementation of ultrafast laser ablation for phonosurgery.
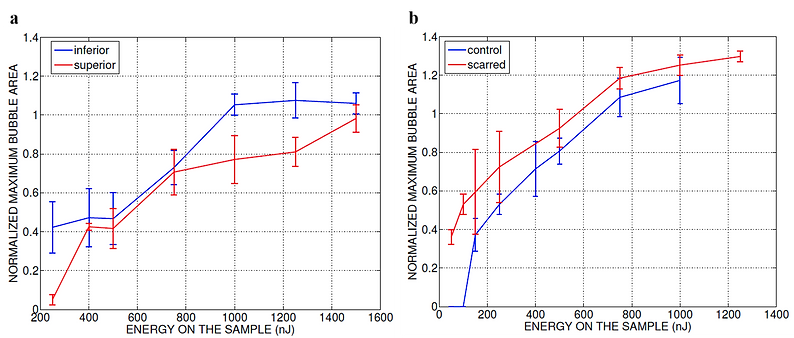
Normalized area of the initial bubbles in the ablated voids at different incident pulse energies 1min after ablation. Each data point represents an average of (a) 15 measurements in five fresh porcine vocal folds and (b) nine measurements in three hamster cheek pouches. The error bars represent the standard deviation of the mean of the samples.
Then, we wrote an review paper on overview of recent advances in bringing unique ultrafast laser surgery tool into the clinic. In this paper, we discussed fundamental mechanisms and limitations of ultrafast laser ablation, novel technique for overcoming these limitations, the current state of clinical applications, and conclude with our recent efforts in developing fiber-coupled probes for flexible ultrafast laser surgery and imaging.

Injection of PEG30 biomaterial into scarred tissue with and without ablation. Fluorescence images of (a) injection without ablation, from left to right: before injection, just after the injection, and after cleaning the tissue surface showing that it was not possible to inject and localize the biomaterial inside the scar tissue. The dashed lines indicate the region of injection. (b) Injection into an ablated void of 2×1 mm2 size after wiping of tissue surface. The fluorescence signal originated from the Rhodamine dye embedded in the biomaterial. In this trial, successful localization of the biomaterial was only possible when injecting into an ablated void. Scale bars are 1 mm
In all of the previous studies, we could ablate upto 100 micrometers deep because there was no enough signal beyond this depth with two-photon based imaging techniques. Thus, we focused on the use of longer excitation wavelengths and three-photon based techniques to improve imaging depth with porcine vocal folds. The experimental, analytical, and Monte Carlo simulation results revealed that third-harmonic generation (THG) at 1552 nm improves the imaging depth in observed in two-photon microscopy at 776 nm from 140 to 420 μm. This value almost doubles the previously reported normalized imaging depths of 3.5 to 4.5 extinction lengths using three-photon-based imaging modalities. Since tissue absorption is substantial at the excitation wavelength of 1552 nm, this study assesses the tissue thermal damage during imaging by obtaining the depth-resolved temperature distri- bution through a numerical simulation incorporating an experimentally obtained thermal relaxation time (τ). By shuttering the laser for a period of 2τ, the numerical algorithm estimates a maximum temperature increase of ∼2°C at the maximum imaging depth of 420 μm. This paper demonstrates that THG imaging using 1552 nm as an illumination wavelength with effective thermal management proves to be a powerful deep imaging modality for highly scattering and absorbing tissues, such as scarred vocal folds.
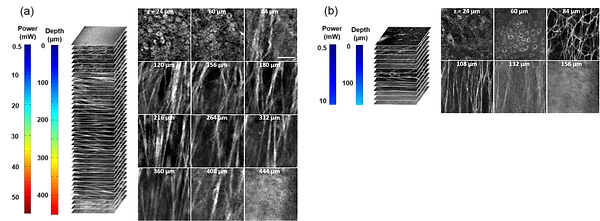
Representative nonlinear optical images of a fresh superior porcine vocal fold. (a) Images of THG showing clear collagen features beyond 400μm using imaging powers in the range of 50 mW. (b) Images of combined TPM and second-harmonic generation indicating maximum imaging depths limited to below 150μm. The scale bar represents 50μm.
Towards utilizing this novel ultrafast laser technology in the clinic, we first presented the optical design of a 9.6-mm diameter fiber- coupled probe for combined femtosecond laser microsurgery and nonlinear optical imaging. Towards enabling clinical use, we successfully reduced the dimensions of our earlier 18-mm microsurgery probe by half, while improving optical performance. We use analytical and computational models to optimize the miniaturized lens system for off-axis scanning aberrations. The optimization reveals that the optical system can be aberration-corrected using simple aspheric relay lenses to achieve diffraction-limited imaging resolution over a large field of view. Before moving forward with custom lenses, we have constructed the 9.6-mm probe using off-the-shelf spherical relay lenses and a 0.55 NA aspheric objective lens. In addition to reducing the diameter by nearly 50% and the total volume by 5 times, we also demonstrate improved lateral and axial resolutions of 1.27 μm and 13.5 μm, respectively, compared to 1.64 μm and 16.4 μm in our previous work. Using this probe, we can successfully image various tissue samples, such as rat tail tendon that required 2-3 × lower laser power than the current state-of-the-art. With further development, image- guided, femtosecond laser microsurgical probes such as this one can enable physicians to achieve the highest level of surgical precision anywhere inside the body.
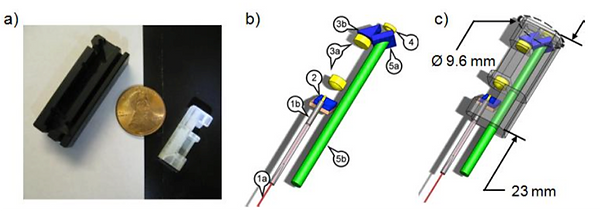
Optical system of the new 9.6-mm probe. a) A photograph of the 9.6-mm probe housing (right) next to the housing of the 18-mm probe (left) showing the reduction in packaged probe size. A US penny is shown for scale. b) The components include: (1a) three meters of PBF; (1b) the fiber collimation assembly; (2) a two-axis MEMS scanning mirror; (3a) a pair of spherical relay lenses with 2.32 × magnification; (3b) a right angle TIR prism; (4) a 0.55 NA aspheric objective lens; (5a) a dichroic mirror; and (5b) one meter of 2 mm core plastic optical fiber. c) The packaged endoscope is shown transparently overlaid with the optical system.
Towards developing precise microsurgery tools for the clinic, we previously developed image-guided miniaturized devices using low repetition rate amplified ultrafast lasers for surgery. To improve the speed of tissue removal while reducing device diameter, here we present a new 5-mm diameter device that delivers high-repetition rate laser pulses for high speed ultrafast laser microsurgery. The device consists of an air-core photonic bandgap fiber (PBF) for the delivery of high energy pulses, a piezoelectric tube actuator for fiber scanning, and two aspheric lenses for focusing the light. Its inline optical architecture provides easy alignment and substantial size reduction to 5 mm diameter as compared to our previous MEMS-scanning devices while realizing improved intensity squared (two-photon) lateral and axial resolutions of 1.16 μm and 11.46 μm, respectively. Our study also sheds light on the maximum pulse energies that can be delivered through the air-core PBF and identifies cladding damage at the input facet of the fiber as the limiting factor. We have achieved a maximum energy delivery larger than 700 nJ at 92% coupling efficiency. An in depth analysis reveals how this value is greatly affected by possible slight misalignments of the beam during coupling and the measured small beam pointing fluctuations. In the absence of these imperfections, self-phase modulation becomes the limiting factor for the maximum energy delivery, setting the theoretical upper bound to near 2 μJ for a 1-m long, 7-μm, air-core PBF. Finally, the use of a 300 kHz repetition rate fiber laser enabled rapid ablation of 150 µm x 150 µm area within only 50 ms. Such ablation speeds can now allow the surgeons to translate the surgery device as fast as ~4 mm/s to continuously remove a thin layer of a 150 µm wide tissue. Thanks to a high optical transmission efficiency of the in-line optical architecture of the device and improved resolution, we could successfully perform ablation of scarred cheek pouch tissue, drilling through a thin slice. With further development, this device can serve as a precise and high speed ultrafast laser scalpel in the clinic.

Optical architecture and resolution of the scalpel. a) A computer assisted drawing (CAD) of the scalpel consisting of 1 – piezo actuator tube, 2 – air core PBF, 3 – collimation lens, and 4 – objective, as overlaid with ZEMAX ray tracing diagram. Blue arrow shows fiber fixation location. b) Measured and theoretical lateral and axial intensity distributions.
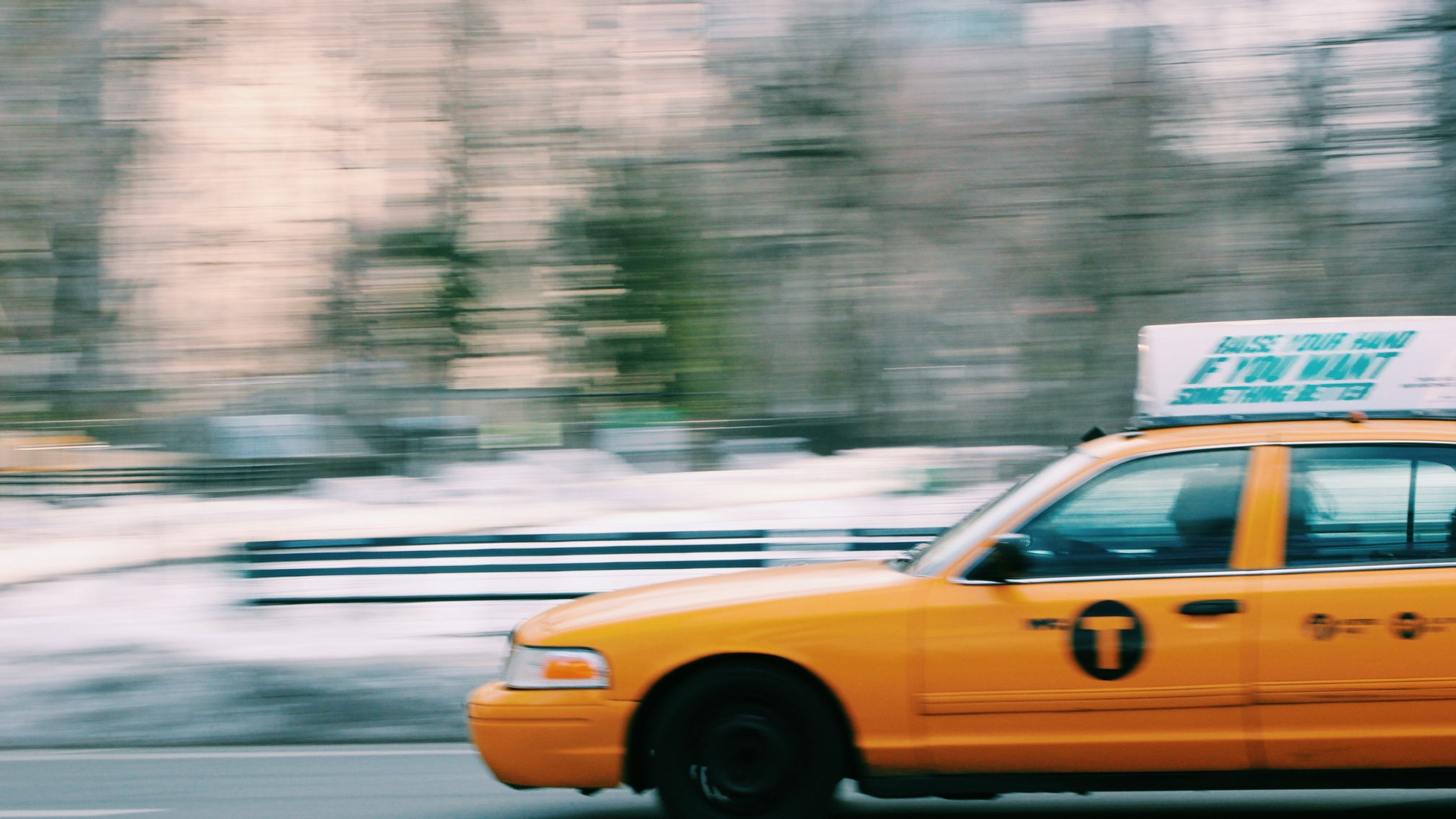
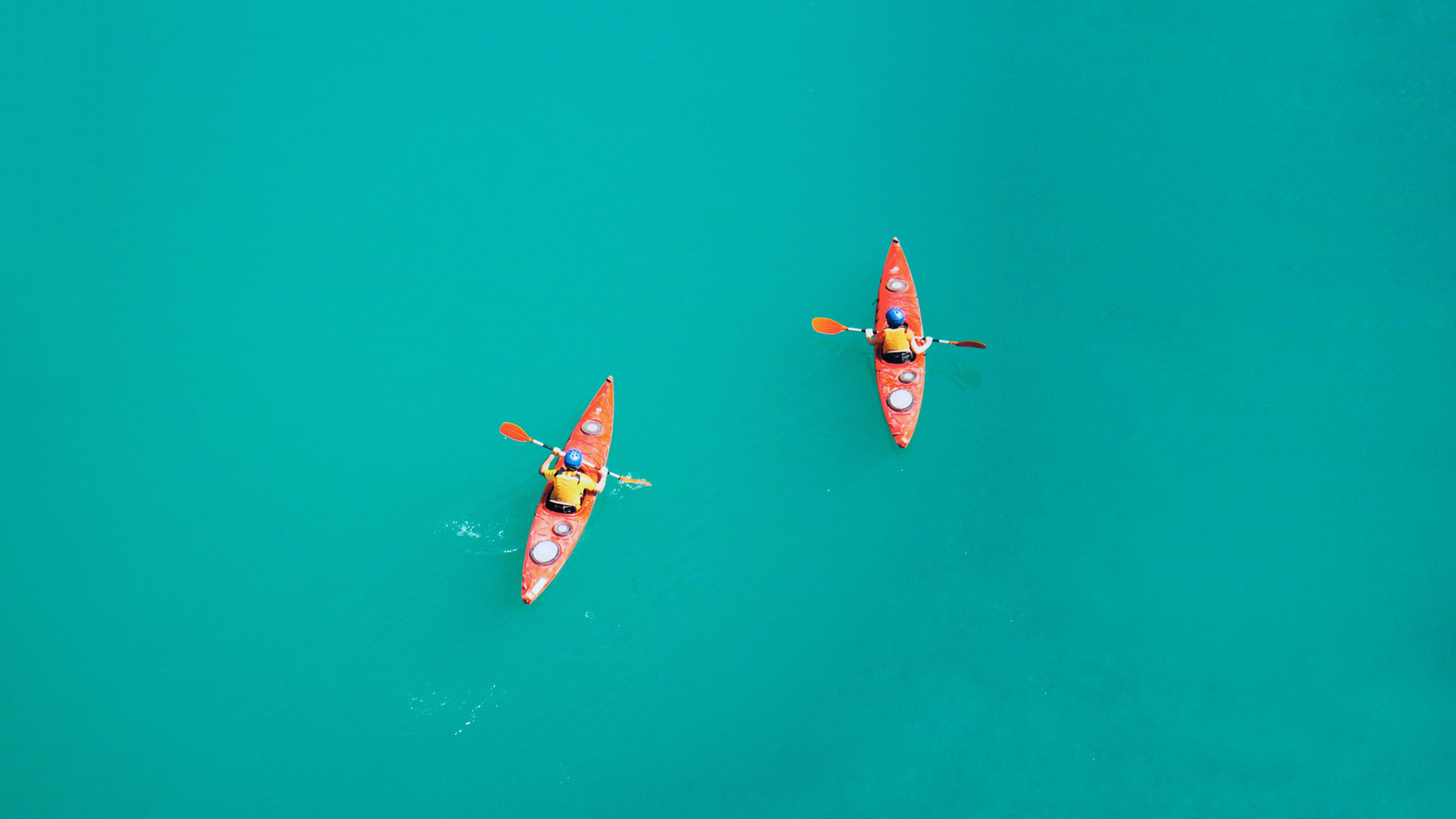
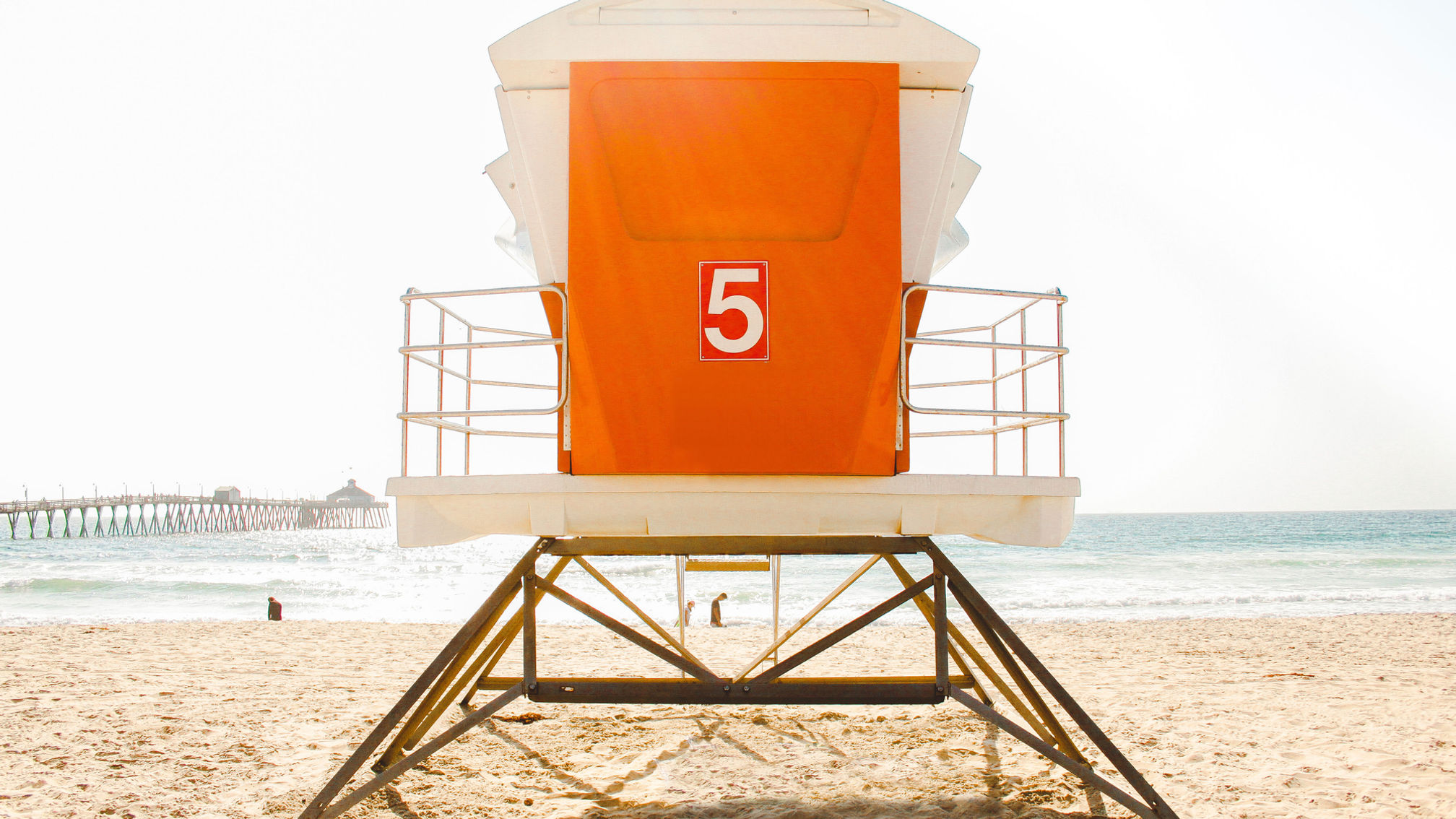
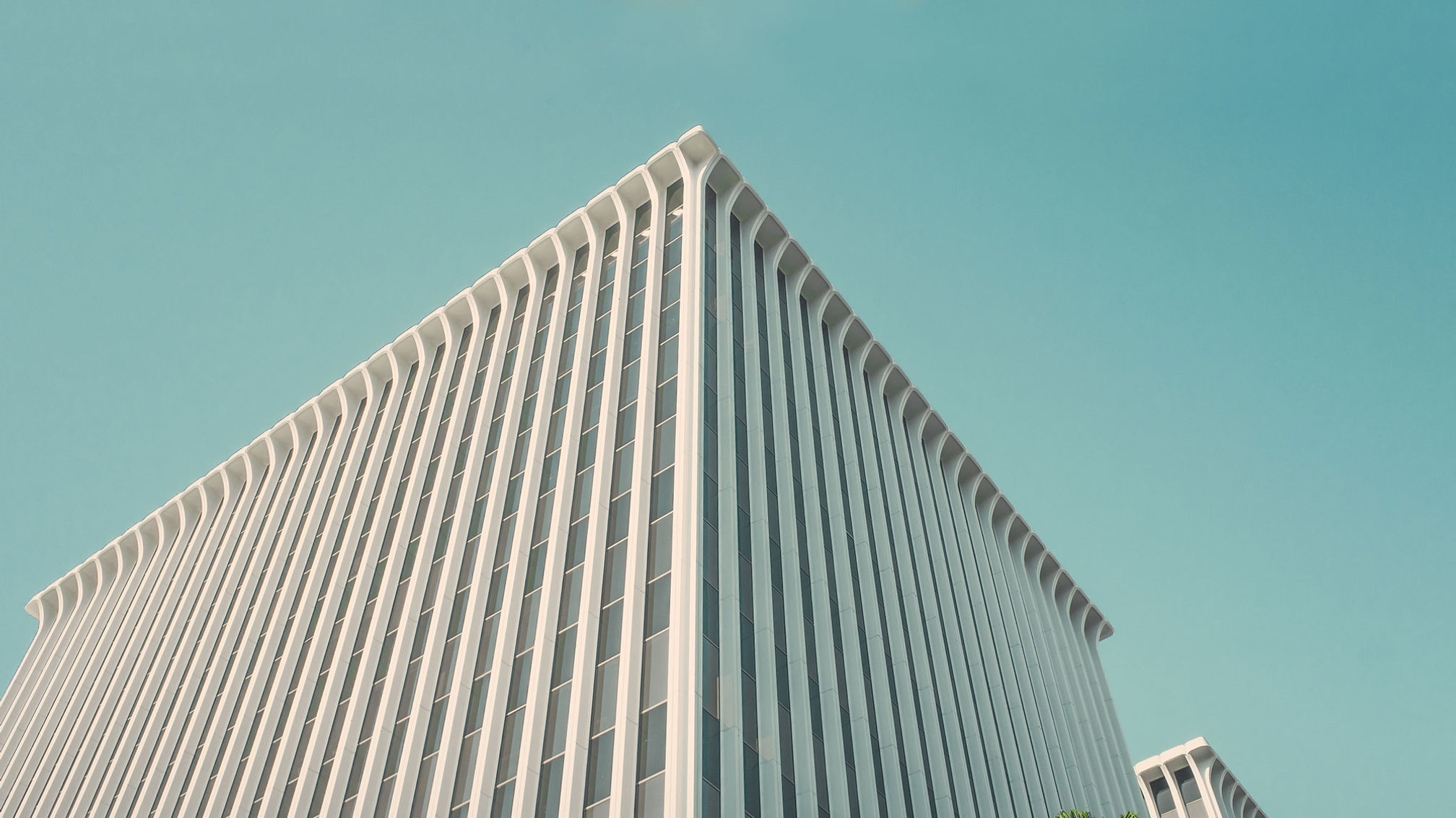
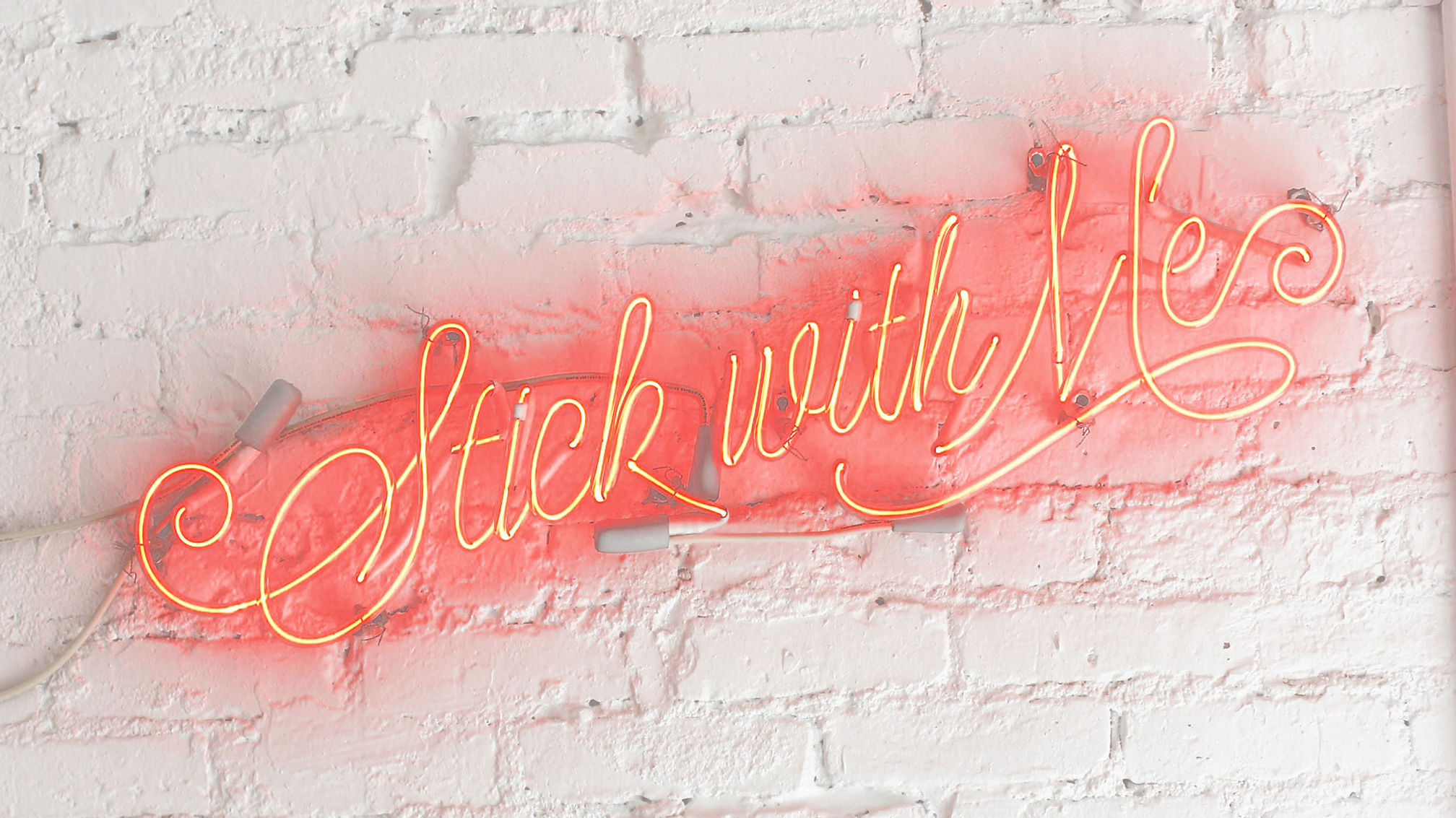
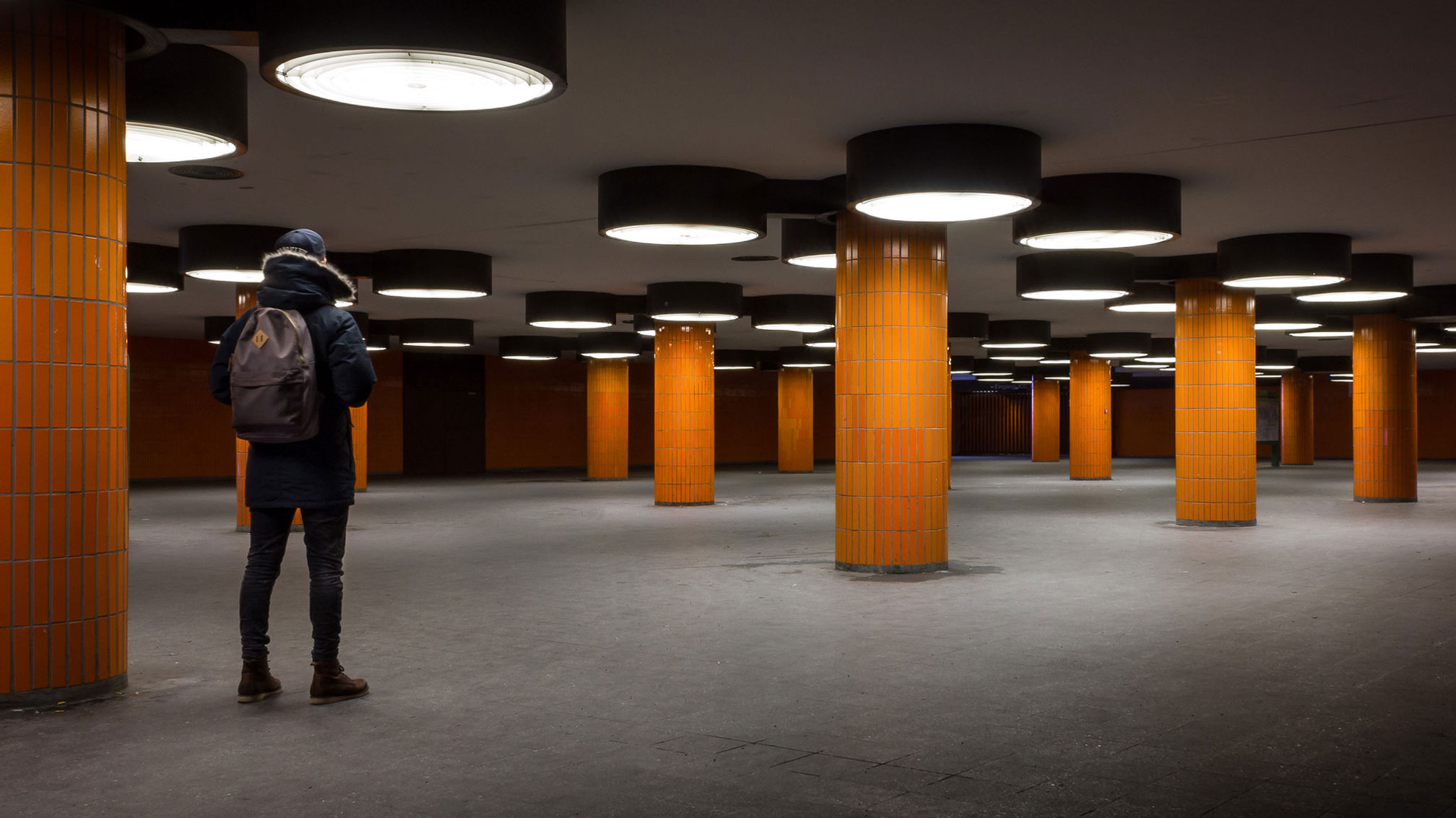
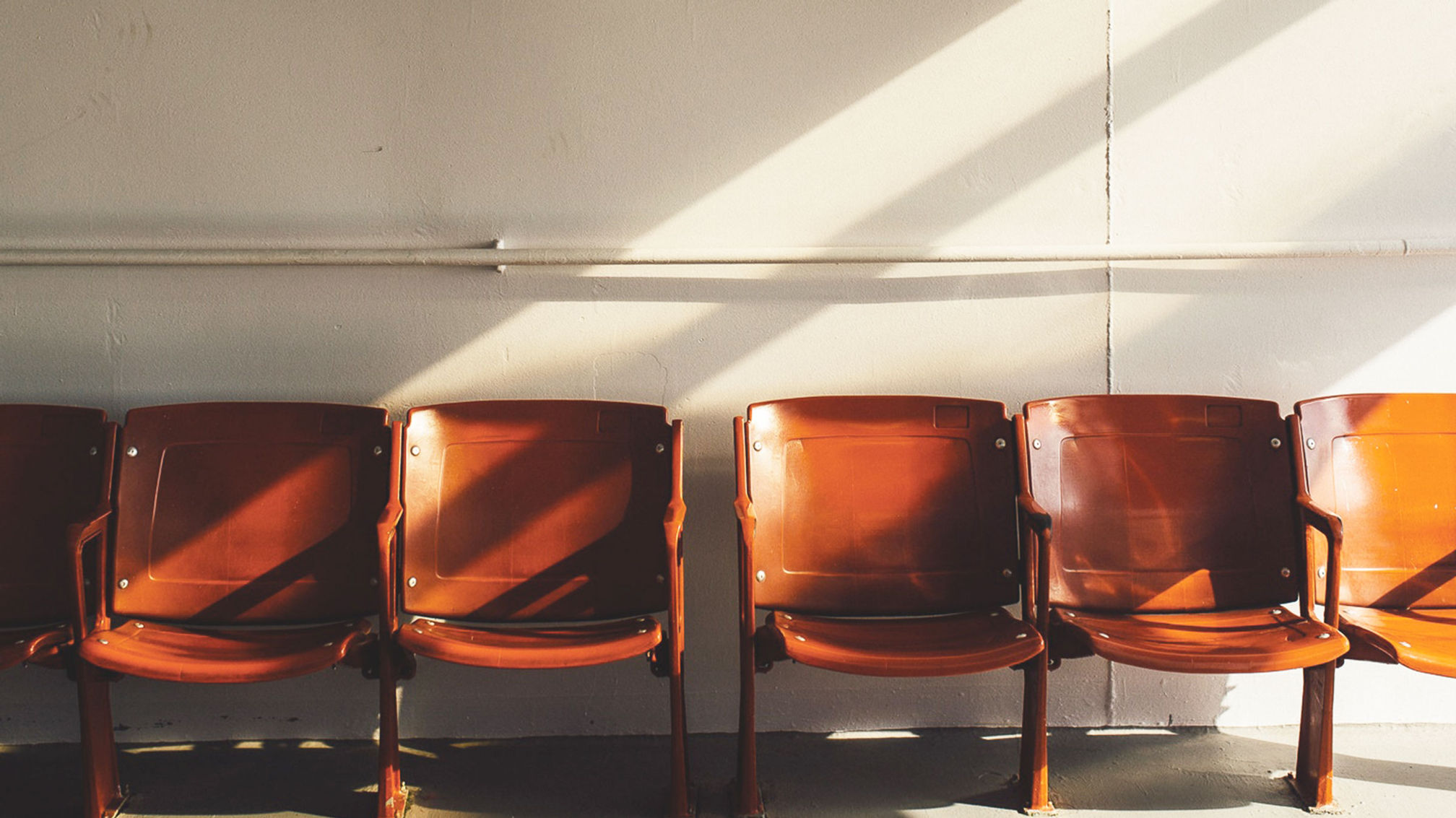
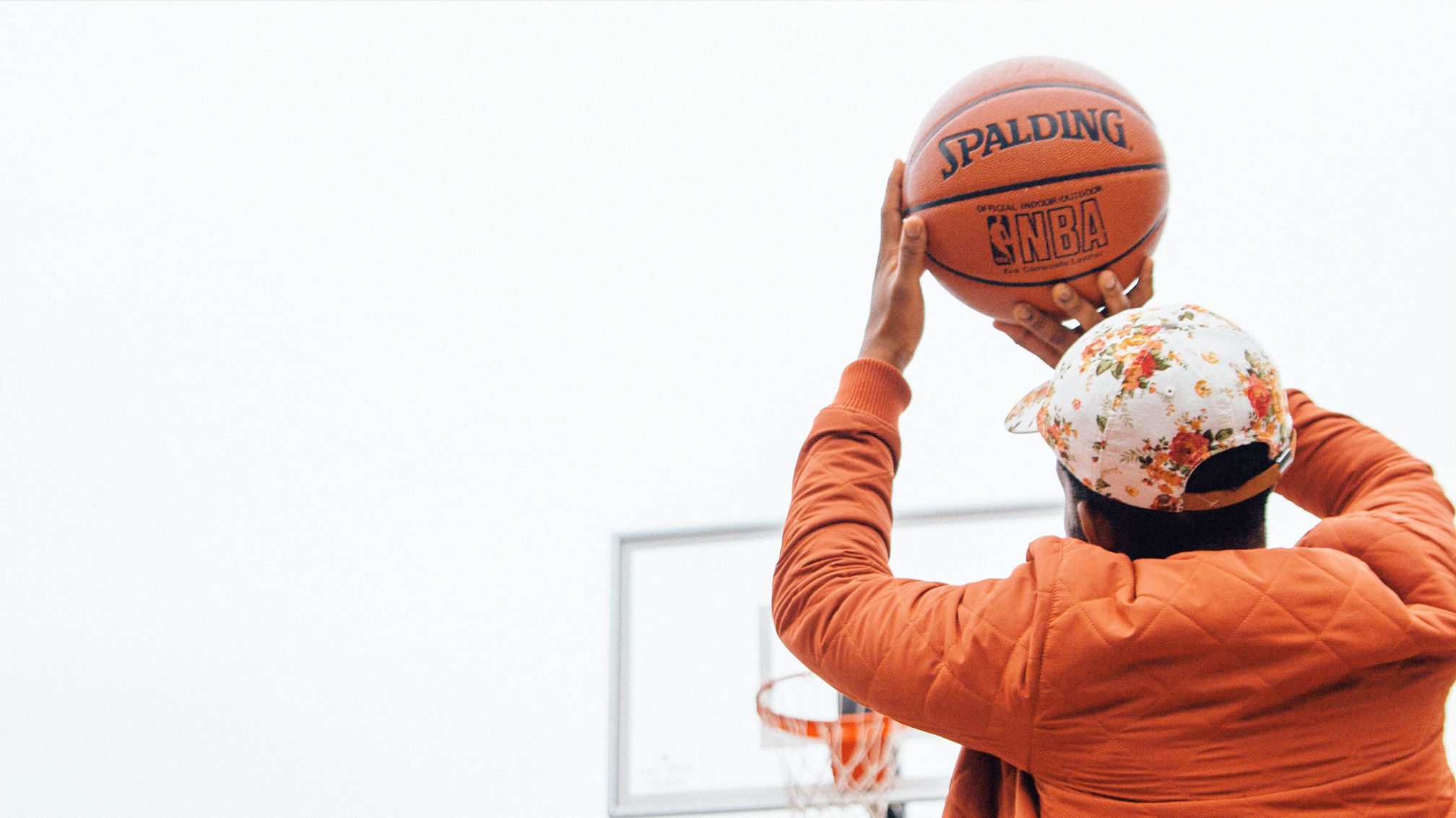